A wide variety of diseases is caused by an error or mutation in our DNA. The consequences of these errors can vary from relatively mild symptoms in essentially any organ to a significantly reduced quality of life and life expectancy. While these diseases are individually rare, there are around 10,000 rare diseases that collectively affect a significant part of the world’s population.
Fixing these genetic errors – sometimes thousands of different ones causing one disease – is the premise of gene therapy, and it’s one of the main lines of research at University Medical Center Utrecht to develop new and better gene therapies.
Strong diagnostics but no treatment
The Human Genome Project kickstarted a lot of research and insights into our genome. Sequencing technology got better and cheaper, and this made diagnosis of genetic diseases possible and easier. As Indi Joore, PhD student developing gene therapies for metabolic diseases at UMC Utrecht explains: ‘We can tell a patient exactly which gene and which enzyme are affected.’
Unfortunately, finding the mutation is not the same as fixing it. Treatment options for many genetic diseases remain extremely limited. Joore: ‘This puts patients in the position where they know exactly what is wrong, but nobody can help them.’
The need for better therapies
Gene therapy, based on genetic engineering, is picking up the slack. ‘Especially since CRISPR technology came, and caused a total revolution in the field,’ Ewart Kuijk remembers. Kuijk is assistant professor at the UMC Utrecht and works in the Regenerative Medicine Center Utrecht, together with Joore.
Genetic engineering technologies like CRISPR-Cas can accurately locate and correct mutations by switching out the nucleotide or supplying a functional copy of the gene. But it’s one thing to modify the DNA of cells in a culture, and quite another to provide a treatment to patients.
‘To be able to translate the lab work into a successful clinical intervention, gene therapy has to consist of three functioning parts: a delivery vehicle, the gene editing therapeutic as cargo and the precise cell-targeting mechanism.”
The trinity of gene therapy
‘To be able to translate the lab work into a successful clinical intervention,’ Kuijk explains, ‘a gene therapy has to consist of three functioning parts: a delivery vehicle, the gene editing therapeutic as cargo and the precise cell-targeting mechanism.’ Researchers at the UMC Utrecht are working on each component of this trinity, aiming to develop genetic editing technology able to target and enter a specific cell type and repair the disease-causing mutation where it matters.
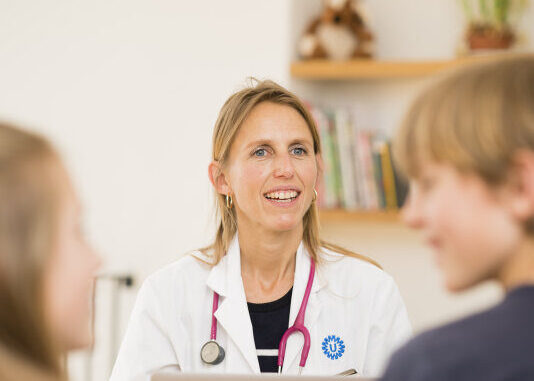
Designing the right cargo
The essential element of a successful gene therapy is the ‘cargo’ of genetic editing tools. The Sabine Fuchs group, that Indi Joore is part of, is developing gene editing tools to cure rare metabolic diseases.
Treating a patient in the lab first
When a patient is diagnosed in the hospital, often the WKZ, a skin or organ-specific biopsy is taken for the exact classification of the disease. ‘When there is sufficient tissue for the clinical procedure, we can use the residual cells to create a disease model of this specific person,’ Joore says.
With this patient-specific disease model, the team can start designing a genetic engineering tool to repair the disease-causing mutation. ‘Once the tool we developed is efficient enough, we check whether it is safe and how we can deliver it to patients. For the liver, the main metabolic organ, fortunately, we only need to repair a tenth of the liver cells to treat the symptoms of the disease.’
“Once the tool we developed is efficient enough, we check whether it is safe and how we can deliver it to patients. For the liver, the main metabolic organ, fortunately, we only need to repair a tenth of the liver cells to treat the symptoms of the disease.”
As tailored as needed, as universal as possible
Designing a gene editing tool for each individual patient is a challenging proposition. A disease might be caused by a multitude of different errors in the same gene or even in different genes. Thus, developing a cure for a single patient could take months or even years, leading to delayed treatment for the patient and high development costs.
A good example for this problem is cystic fibrosis, an illness that can be caused by 2,000 different mutations in the same gene. Ewart Kuijk explains: ‘Each mutation requires a specific strategy, a specific design of your prime editors and base editors that have specific efficiencies. Instead of trying to repair the gene causing the disease, an alternative could be to insert a functional copy of the gene into a different spot in the genome. This would treat the disease, even if it doesn’t fix the mutation.’
Targeting the right cell type
The second element of a gene therapy is delivering the gene editing tools to the cells where it counts.
Specific targeting is better
Ewart Kuijk and his colleagues are working on making delivery methods as cell type-specific as possible, aiming to only target the relevant cells. Kuijk: ‘If you use non-specific delivery for a treatment, it will end up in many cells that may not include the cells that need it the most. With cell type-specific delivery only the disease-causing cells can be targeted and the concentration of the therapeutic can be kept low. To do this, we look for cell type-specific surface markers, used in cell-to-cell recognition.’
Targeting has to include all relevant cell types
Finding the right markers is easier said than done, as some diseases are not contained within a single cell type. ‘Cystic fibrosis, for example, is most noticeable in the lungs, but the disease also affects the intestines and the pancreas. Thus, multiple different delivery strategies would need to be developed to completely cure the disease,’ Kuijk adds.
Targeting cells for long-term effects
Another factor to consider is the regenerative capacity of tissues. ‘If there is a genetic defect causing the enterocytes in the intestine to not function properly,’ Kuijk starts, ‘we may be able to find a way to deliver a treatment to those cells. But enterocytes are differentiated cells of the intestine, which means that after one week, those cells are all shed into the lumen and flushed down the toilet. In other words, we need to think about which cells to target. In this example you would need to target the intestinal stem cells to have long-lasting effects.’
The delivery vehicle
The third element of a successful gene therapy is the delivery vehicle.
LNPs deliver in the liver
Joore: ‘Since the development of the COVID vaccine, there has been a huge technological boom in liquid nanoparticles or LNPs containing modified RNA.’ Research has shown that intravenously injected LNPs accumulate in the liver, making them an optimal delivery vehicle for the treatment of liver-dominant metabolic diseases. ‘Therefore,’ Joore continues, ‘we are now working on optimizing LNPs for prime editing, looking how we can best package the RNA and code the most efficient tools.’ The group recently started their first pilot experiments in mice, testing the efficacy of prime editing using LNPs in vivo.
Going beyond the liver
However, if the disease requires a different organ to be targeted, bypassing the liver becomes necessary to ensure efficiency and reduce off-target effects and liver damage. As Kuijk puts it: ‘You then want to bypass the liver because otherwise the liver will eat up all your precious particles, and that could come at the cost of off-target side effects. And if you can use less particles for your gene therapies, that also means that you have less risk of adverse immune reactions.’
Utilizing viruses for gene therapy
More cell type-specific delivery vehicles that are already being used in patients are viruses. In nature, viruses invade cells, hijacking them to replicate. This mechanism can be modified to serve as a therapy. Kuijk: ‘Lentivirus vehicles, for example, are already being used to treat haematological diseases by shuttling in a healthy copy of a gene.’
However, the use of viruses for cell-type specific delivery does not come without risk. They can cause immunological reactions and their integration into the cell’s genome may cause cancer. So, is it possible to get the benefits of a virus without the downsides?
Introducing virus-like particles
Ewart Kuijk thinks it is. ‘There are virus-inspired particles. These look like a virus, but they are produced in cell lines, that you trick in producing something that looks like a virus from the outside, but inside you put gene editing tools like base and prime editors instead of a virus genome. And you use that as a Trojan horse to deliver your cargo into the cells of interest.’
In developing these virus-like particles, Kuijk benefits from the close collaboration with researchers in the RMCU. ‘Jeffrey Beekman has an organoid assay for cystic fibrosis with a very strong readout for whether the mutation that causes the disease is repaired or not. He uses it to test existing drugs, but I now have a grant to use this assay to see if my virus-like particles are also able to reach and fix the mutation.’
A workable future for gene therapy
The field of genetic engineering is evolving in many ways at the same time, slowly but surely moving towards more clinical applications. However, to make gene therapies part of the ‘standard of care’, the drug approval process must undergo a shift in mindset.
A toolbox full of approved technologies
Both Ewart Kuijk and Indi Joore see the need for a more universal testing method in the development of new therapeutics. Joore: ‘In the future we should have a gene therapy toolbox with different delivery vehicles, gene editing tools and cell-type specific targeting systems that can be combined to create a personalised treatment for each patient.’
This approach would require a more universal testing procedure in which each component is evaluated separately and ready to be assembled in a variety of combinations. Kuijk adds: ‘At the moment, each new therapy has to undergo thorough testing in cellular models, animal experiments and clinical studies before it can be approved. Therefore, finding a solution to universalise testing could save a lot of money and time, making therapies more accessible to patients.’
In the case of rare diseases, this pre-approved genetic toolbox would take away a big hurdle in developing treatments. Joore: ‘No one can finance the required studies and research infrastructure for small patient groups or individuals. But we work with patients that have no alternative to being sick, so there needs to be a solution.’